The first of The Three Little Pigments is green. This is the story of chlorophyll.
Leaves are the biosphere’s solar panels. If this is so, why are they green and not black? Science is better at answering ‘how’ questions than ‘why’. Here’s a possible explanation for how leaves came to be green.
It’s better for a solar panel to be black because it absorbs light more or less equally from the blue to red end of the visible spectrum. Some people think the pigments of the earliest photosynthetic lifeforms absorbed light across most of the middle range of the spectrum. Organisms that take light over these wavelengths would appear to the eye as dark purple in color – not so far from the solar panel ideal.
Occupation of the central wavebands would create a niche. Organisms with pigments that could use light at the extreme red and blue ends of the spectrum could make a living. Chlorophyll has just such a light absorption profile and appears green to the eye.
For some reason, the green photosynthesizers prevailed during evolution and became the ancestors of all the vegetation that surrounds us today.
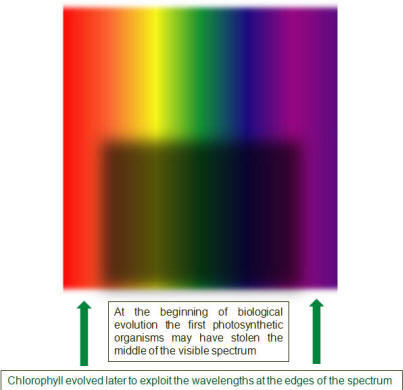